Onkyo (DES-Integra) y Pioneer por añadido ya son chinas. Otras más
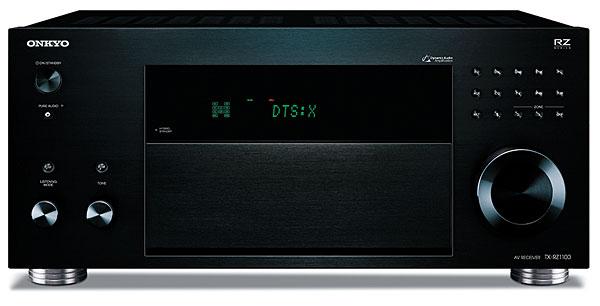
Onkyo, su historia, su fundación, luego a manos de Toshiba, su adquisición de Pioneer, su compra por parte de Gibson y ahora pasa a ser otra compañía más, otra más penosamente en manos chinas.
¡Que lamentable!
TCL el fabricante chino de TVs baratas compró a Onkyo de la atribulada Gibson brands.
Y TCL ahora va por parte de Philips y ya es dueña de las marcas Alcatel y Blackberry de teléfonos.
Chinese technology Company TCL is set to take over the Pioneer and Onkyo brands currently under the management of US Company Gibson brands, they are also in talks with Philips over their audio range according to sources.
The move is set to be a major blow for Gibson Brands who are facing a grim future with debt as high as A$707 million due within months.
Como casi toda cosa china, auqneu muchos se molesten algunas además de baja calidad tienen un nulo penetramiento de marca, tuvo que pagar por derechos de marca Toshiba ya que la marca propia de TCL HiSense resultó muy mala y los consumidores la rechazaron en EUA. TCL afima que invertirá en Onkyo y Pioneer. Habrá que ver.
Hace 15 años...
Industry News 15 Years Ago
Universal Music Makes Commitment In Producing DVD-Audio
Universal Music Group (UMG) has announced their commitment to make music available on DVD-Audio. Beginning with twenty United States releases slated for the second half of 2003, UMG plans an ongoing program to make a combination of best-selling artists and core catalog titles available on DVD-Audio in major markets worldwide. "Universal has always played a leading role in the development and implementation of new technologies and formats," states Larry Kenswil, president, eLabs, Universal Music Group. "Over the past five years DVD has established itself as one of the fastest growing formats. The huge penetration of DVD makes DVD-Audio a natural progression for music lovers who are already owners of DVD players, taking them from the familiar world of stereo to the 21st Century world of multi-channel surround sound."
Universal Music Makes Commitment In Producing DVD-Audio
Universal Music Group (UMG) has announced their commitment to make music available on DVD-Audio. Beginning with twenty United States releases slated for the second half of 2003, UMG plans an ongoing program to make a combination of best-selling artists and core catalog titles available on DVD-Audio in major markets worldwide. "Universal has always played a leading role in the development and implementation of new technologies and formats," states Larry Kenswil, president, eLabs, Universal Music Group. "Over the past five years DVD has established itself as one of the fastest growing formats. The huge penetration of DVD makes DVD-Audio a natural progression for music lovers who are already owners of DVD players, taking them from the familiar world of stereo to the 21st Century world of multi-channel surround sound."
http://www.enjoythemusic.com/news/
Aletheia A-26G2: Ajuste final. Primer escucha 2
Aletheia A-26G2
Diseño sellado, woofer 6.5", tweeter con radiador de doble anillo. Red de cruce con componentes de elevada calidad Mundorf, de Alemania. Cable interno GoldKabel HighFlex Anthrazit. Soldadura con plata Groneberg. Baffle sellado internamente con recubrimiento a base de polimeros.
Diseño sellado, woofer 6.5", tweeter con radiador de doble anillo. Red de cruce con componentes de elevada calidad Mundorf, de Alemania. Cable interno GoldKabel HighFlex Anthrazit. Soldadura con plata Groneberg. Baffle sellado internamente con recubrimiento a base de polimeros.
Lo sabemos, un video en Youtube o en el web no dice nada ni da fe, ni es aval del desempeño de un sistema o equipo particular, sí. ¡Pero vaya! Como sea es entretenido compartir y son regularmente bien recibidos.
Disculpen la solvencia técnica del video, acaba de realizarse el ajuste final al diseño por lo que reina la emoción y no se pudo resistir más el deseo de compartir.Se grabó con teléfono LG Q10. :)
Este modelo queda ya en disponibilidad comercial inmediata, bajo pedido.
Sistema NuPrime DAC-9 y dos STA-9. Cabelado Goldkabel. Acondicionador Aletheia SauberStrom.
Sistema NuPrime DAC-9 y dos STA-9. Cabelado Goldkabel. Acondicionador Aletheia SauberStrom.
Aletheia AV SauberStrom: Acondicionador eléctrico Hi-Fi
Aletheia AV SauberStrom
Video demostración de desempeño
Espero se entienda el video, de todos modos explico un poco: A una misma linea eléctrica se conectó un multímetro solo para fines de comprobación de voltaje y dos cables de alimentación. Se dejó preparado un cable de alimentación directo para el analizador de ruido eléctrico de banda ancha Entech, el otro cable de alimentación va al acondicionador SauberStrom y desde el banco 1 del acondicionador se dejó preparado otro cable para conectar el medidor de ruido Entech en la segunda etapa de la prueba. Primero se conectó directamente el analizador de ruido eléctrico de banda ancha Entech ajustando el nivel de ruido a 100, que es como indica el fabricante. A continuación se desconectó del cable directo y se conectó al cable procedente del banco 1 del acondicionador Aletheia SauberStrom. La diferencia en el valor del medidor pasó de 100 estando conectado directamente a la linea eléctrica a 8~5 estando ya conectado al acondicionador SauberStrom, así mismo la disminución en el ruido audible es evidente.
www.aletheia-av.com
www.facebook.com/aletheia.av/
¿Te esperarías algo así en unas bocinas de 6000 Euros?
Una nueva entrega de "Bonito por fuera ¿Bueno por dentro?"
La imagen corresponde al crossover de unas bocinas que suenan bien, eso no se pone en juicio y que se venden en 6000 Euros.
¿Acaso por 6000 Euros no podría el fabricante poner más empeño en calidad de partes, terminado de crossover y calidad de cajón y regalarle más satisfacción al comprador?
Es un fabricante nórdico, la bocina tiene nombre de mujer y canción de Fleetwood Mac.
Curiosidades. Google, modas y falsos entendidos.
Buscando en Google "music listening" aparecen estas imágenes.
Datos curiosos que me hacen pensar sobre como Google manipula resultados para ir acorde a intereses comerciales:
Datos curiosos que me hacen pensar sobre como Google manipula resultados para ir acorde a intereses comerciales:
- Nadie está escuchando realmente música en las imágenes, están conectados a un aparato y audífonos
- No hay en la primer página de resultados un grupo musical, un músico, una orquesta, un cantante ni una sala de conciertos o espacio similar, nada que realmente y de primera mano cree o esté ligado a la creación de música.
- Todos, todos solo tienen audífonos.
Seguramente solo suspicacias de mi parte pero me pareció peculiar.
Escuchar música no es escuchar audífonos y ni un Hi-Fi en casa o auto es estar en vivo frente a la música misma, lo otro es escuchar Hi-Fi o audio
Gran artículo sobre música, la escucha humana y el audio
Espero poder traducirlo en breve, me parece concreto y muy informativo.
El artículo original en: http://www.silcom.com/~aludwig/EARS.htm
El artículo original en: http://www.silcom.com/~aludwig/EARS.htm
Music and The Human Ear
This section contains information on the softest and loudest sounds we can hear, the range of frequencies we can hear, subjective vs. objective loudness, how we locate the source of a sound, and sound distortion. This section focuses mainly on the ear itself, but the brain is an integral part of the human hearing system. A separate section considers the function of the brain in more detail.
The human ear is a truly remarkable instrument. At one point in my life I designed Electronic Counter Measures (ECM) systems for the U. S. military. The primary function of an ECM system is to detect an enemy before he (it's rarely a she) detects you, for self-defense. It is interesting to compare the characteristics of a good ECM system and human hearing:
Comparison of characteristics
Characteristic
|
Good ECM system
|
Human hearing
|
Directional coverage
|
All directions
|
All directions
|
Source location accuracy
|
Within 1-5 degrees
|
About 5-degrees
|
Ratio of highest to lowest frequency (bigger the better)
|
20 : 1
|
1000 : 1
|
Ratio of strongest signal to weakest (the bigger the better)
|
Million : one
|
32 trillion : one
|
Human hearing is a superior defensive system in every respect except source location accuracy. Note: Jourdain (page 23) states that human accuracy is 1-2 degrees in azimuth.
In contrast, a military system designed for communications (rather than detection) would typically have a much smaller ratio of highest-to-lowest frequency, no source location capability, and often a narrow directional coverage. For human communication a frequency ratio of 10:1 and a ratio of strongest to weakest signal of 10,000:1 would suffice. The far larger actual ratios strongly imply a purpose other than communication.
All of this tells me that the ear evolved primarily for self-defense (or perhaps hunting, as one reader pointed out), and language and enjoyment of music are delightful evolutionary by-products. A defensive purpose also suggests some direct hard-wiring between the ears and primitive parts of the brain, which may account for the powerful emotional impact of music - and its virtual universality among human cultures. A few years after writing this paragraph I found the very interesting book This is Your Brain on Music which confirms the speculation on wiring to primitive parts of the brain, but argues that music has a definite evolutionary function.
Acknowledgment: a good part of the material in the remainder of this section is derived from an excellent book The Master Handbook of Acoustics by F. Alton Everest, and from the chapter he contributed to the Handbook for Sound Engineers. See references. These sources also contain much additional interesting material. David Worrall has posted his course notes of Physics and Psychophysics of Music on the web, which includes an informative section on the physiology of hearing. A series of tutorial papers on hearing and other related topics has also been posted by HeadWize.
Sound pressure level (SPL) is given in dB SPL. This is a scale that is defined such that the threshold of hearing is close to 0 dB. The threshold of pain is about 135 dB. This is a logarithmic scale where power doubles for each 3 dB increase; the 135 dB difference between the thresholds of hearing and pain means the power doubles about 45 times - an increase of 32 trillion (32x1012) in the power level. This is an incredible dynamic range, and totally blows away anything human engineers are capable of creating. (Actually in a Dec 99 Newsgroup post Dick Pierce states that B&K 4138 microphones have a dynamic range of 140 dB, so I was underrating human engineers). At the low end of the range the ears lose function due to background noise. At 0 dB SPL noise created by blood flow in the ear is one source. It is shown elsewhere that the noise of molecules colliding with the eardrum is not far below this level. At the threshold sound level of 0 dB SPL Everest states that the eardrum moves a distance smaller than the diameter of a hydrogen molecule! (Correction! it is the stereocilia in the inner ear that move this much, not the eardrum). At first I was incredulous when I read this, but it is consistent with the change in diameter of the balloon example used in the previous section. For a 0 dB SPL the change in balloon diameter is 6x10-10 inches, which is about 1/10 of the diameter of a hydrogen atom. The sensitivity of the ear is truly mind-boggling.
Pressure is an objective physical parameter. The relationship of SPL to the subjective sensitivity to sound is discussed below. The human ear is most sensitive in a band from about 2,000-5,000 Hz. This is an important region for understanding speech, and could be construed to imply that hearing evolved to match speech. However, did the ear evolve to be sensitive to the speech frequency band, or did human speech evolve to match the band where the ear is most sensitive? (I read somewhere that babies cry in the frequency band where the ear is most sensitive). As measured by Voss and Allen, a typical eardrum absorbs about 75% of the incident sound energy at 5 kHz. The sensitivity vs. frequency behavior has a fair resemblance to the response of a piston load matched to the impedance of air, as shown in the physics section. Music levels vary from about 50 dB for quiet background music to maybe 120 dB for a very loud rock band. Subjectively, a 2-3 dB change in sound level is barely perceptible; if someone asks you to "turn up the volume a little," you will probably increase the sound by at least 3 dB. (Note that if you have a 100-Watt amplifier and it doesn't play loud enough, you need a 200-Watt amplifier to turn up the volume 3 dB. This can get very expensive very quickly). Interestingly there were some ABX test results on the web which indicate that a 0.3 dB difference in level can be detected (link no longer exists). However the test procedure allows switching between the two levels as much as you want before making a decision, and the test used pink noise for the sound. You can hear what a 3 dB difference sounds like yourself with sound files in the sound demo section.
A full orchestra can also hit a sound level of 110 dB and more, and then play a quiet passage at 20-30 dB. To reproduce this faithfully requires a recorded sound source capable of covering this 80+ dB dynamic range. (Everest quotes one researcher who claims a 118 dB range is required). A vinyl record is good for about 50-70 dB; a standard compact disc with 16-bit encoding can cover a 96 dB range, and the 24-bit DVD disk format a 144 dB range - in theory. Real D/A converters tend to be noise limited to a somewhat lower range.
A problematic aspect of music for a sound system designer is that there are brief transients ("spikes") in sound level that far exceed average power levels. Usually people talk about average, or root-mean-square (RMS) power. RMS power is really only important with respect to the generation of heat. In my opinion, peak power is far more important, since this is when a speaker could be driven into a non-linear region, and when an amplifier would clip. These two effects are major causes of distortion. Using Cool Edit 96, I recorded 10-20 second segments from Talking Heads "Burning Down the House," Diana Krall "All or Nothing at All," and Shostakovich Symphony #5. I then processed the cuts in Matlab, to generate the outputs of a 3-way crossover. The crossover frequencies are 300 and 3000 Hz. Both 1st order Butterworth and 4th order Linkwitz-Riley filters were modeled. Finally I calculated the average and peak power in each driver band, with results as shown in the tables below.
Average Power
Driver
|
Talking Heads
|
Diana Krall
|
Shostakovich
| |||
1st order
|
4th order
|
1st order
|
4th order
|
1st order
|
4th order
| |
Tweeter
|
7%
|
3.4%
|
4%
|
1.6%
|
14%
|
4.4%
|
Midrange
|
28%
|
22%
|
32%
|
25%
|
66%
|
64%
|
Woofer
|
65%
|
66%
|
64%
|
60%
|
20%
|
4.6%
|
Peak Power
Driver
|
Talking Heads
|
Diana Krall
|
Shostakovich
| |||
1st order
|
4th order
|
1st order
|
4th order
|
1st order
|
4th order
| |
Tweeter
|
18%
|
13%
|
53%
|
15%
|
15%
|
8%
|
Midrange
|
45%
|
35 %
|
83%
|
89%
|
53%
|
55%
|
Woofer
|
81%
|
88%
|
40%
|
31%
|
16%
|
4.3%
|
All powers are shown as a percentage of the same quantity in the unfiltered music. Note that the average power for the Butterworth adds to 100%, but the Linkwitz-Riley adds to less than 100%. The voltage output of a Linkwitz-Riley coherently adds to unity, but the power addition is less than unity. The peak power is obtained by computing the time-domain waveform of the signal output by the crossover. Then the peak value is found. Typically the peaks occur at different times for the tweeter, midrange, and woofer, so there is no physical significance to the sum of the three powers in this case. The startling result is that by far the greatest demands on peak power are in the midrange for the Krall and Shostakovich. The 4th order reduces the demands in the high and low bands, but there is little difference in the mid-band. Only the Talking Heads cut has a greater demand in the bass. It is also quite significant that even though the average tweeter power is low, the peak tweeter power is not all that much lower than other bands, and in fact is greater than the woofer in some cases!
When I play the Talking Heads cut, my CLIO sound measurement system shows a peak sound level of 100 dB SPL in the room, and an average of around 95 dB. Judging from the oscilloscope connected to the amp outputs, the average amplifier output power appears to be about 17 watts. The ratio of peak power to RMS power was 40:1, 40:1 and 30:1 for the Talking Heads, Diana Krall, and Shostakovich cuts respectively. Therefore, for 17 watt RMS, the peak power demands are on the order of 700 Watts. This indicates that either my amps can put out peaks much higher than their rated power (possible, but I'm not sure), or they are clipping. There are demo files In the sound demo section which simulate clipping by tube and solid-state amplifiers. For more on this subject see the section on amplifier distortion.
Jourdain (page 41) states that an orchestra produces 67 watts of acoustic power at full blast. Loudspeakers have efficiencies on the order of 0.5 to 2% converting electrical power to acoustic power. Even at 2% efficiency this implies that well over 3,000 watts of electrical power would be required to duplicate this sound level. Of course an orchestra plays in a large auditorium, and no doubt less power is needed for a small room. This still indicates that power requirements should not be underestimated.
A major criterion of a good sound system is its frequency response. The usual frequency range considered "hi-fi" is 20-20,000 Hz. These sample tones are audible with good loudspeakers or headphones, but many computer speakers will not reproduce them at all: a 100 Hz tone, (12 kb wav file) and a 10,000 Hz tone (44 kb wav file). Yesterday I did a test using the very accurate signal generator built into my CLIO system. I can clearly hear, and certainly can feel, a 10 Hz tone. My sound system totally poops out below 10 Hz, so I can't test any lower than that. The lowest notes on organs and pianos are 16.4 and 24.5 Hz respectively. Testing at the other extreme, as a 61 year-old male (when I originally wrote this) I can hear a 13,500 Hz tone, but no higher. (It is generally agreed that women are more sensitive to high frequencies). However, good high frequency response is required to produce sharp transients, such as a snap of the fingers. I performed a test using a Ry Cooder CD, "Talking Timbuktu." Track 10 on this disk has some very sharp transients that just leap out at you from a good sound system. My pre-amp has a filter that cuts off frequencies above 12,000 Hz. With this filter in, the transients limp out rather than leap out. This shows that even though I cannot hear a pure tone in most of the range of frequencies cut out by the filter, I can clearly hear the difference in the sound quality of the transients. I repeated this test recently (at age 67) with a segment of this cut recorded as a .wav file, and digitally processed with a 12kHz filter. This time the test was a double-blind ABX test, and I can't reliably detect any difference (I can still hear a 13,000 Hz tone). I now doubt the validity of the earlier test. See the discussion on high frequency tests in the section on sound demos.
James Boyk at Caltech has posted an interesting paper on the frequencies generated by musical instruments between 20kHz and 102 kHz! He also cites a paper that states that people react to sounds above 26 kHz even when they cannot consciously hear the sound. Jourdain (page 42) states that sound can be heard up to 40 kHz if sufficiently loud (A knowledgeable reviewer of the book is skeptical about this claim. Unfortunately the link to the review no longer works).
The ear tends to combine the sound within critical bandwidths, which are about 1/6 octave wide (historically thought to be 1/3 octave). This has led to the practice of averaging frequency response over 1/3 octave bands to produce beautiful-looking frequency response curves. In my opinion this is misleading. Suppose a loudspeaker has a bad dropout (very weak response) over a narrow frequency range; the dropout will be totally obscured by averaging. But when a musical instrument plays a note that just happens to fall in the dropout notch, you will not be able to hear the note. See the example of a warts-and-all response (28.2 kb) vs. a 1/3 octave smoothed response (24.5 kb) from my final system measurements section. Since we can barely hear a 2-dB difference in sound level, it is reasonable to accept ±2 dB as an excellent level of performance for frequency response. In fact this is impossible to achieve in the real world, due to room acoustics. (see the section on room acoustics). Personally I would say a more-or-less practical goal for a sound system installed in a room is a frequency response ±5 dB from 200-20,000 Hz, and maybe ±10 dB from 10-200 Hz. It is also worth noting that the ear itself has a quite variable frequency response, as shown by measured data on head-related transfer functions, and as discussed in the next section.
What is the minimum audible change in frequency? I created two .wav files: case #1 was a series of 1/2 second tone bursts, all at a frequency of 800 Hz; for case #2 the bursts alternated between 800 and 805 Hz. I can reliably distinguish between these two cases in a double-blind test. This difference in frequency is less than 1/100 of an octave. I could also distinguish between 400 and 402 Hz. According to Jourdain (page 18) this is about normal for a young person; at age 61 I'm not supposed to be able to detect a difference of less than about 8 Hz at 400 Hz. But I can. (I repeated this test at age 67, and I still can do it). Sample files are described in the sound demo section. An interesting detail is that tone bursts that start and stop abruptly are easier to discriminate than bursts with a fade-in fade-out. I don't know if this is simply a timing issue, or if the brain is making use of the higher Fourier transform sidelobes that occur for a square window (the spectrum for a tapered burst is extremely narrow, the square burst spectrum has extensive sidelobes about 40 dB below the peak).
For music the audio spectrum is divided into discrete notes. A brief discussion of the interesting subject of musical scales is given in a separate section.
SPL is an objective measurement of sound pressure, or power in watts, and is independent of frequency. In 1933 Fletcher and Munson of Bell Labs did a study that showed that subjective sound levels varied significantly from the SPL level. That is, when two tones were played at the exactly the same SPL level, one sounded louder than the other. And the results were very dependent on how loud the tones were to begin with. This is illustrated by the set of Fletcher-Munson curves [102 Kb]. The vertical axis is the objective SPL sound level. Each of the curves in the graph represents a constant subjective sound level, which are in units called "phones." The lowest curve is the minimum audible level of sound. As noted above, the ear is most sensitive around 2-5 kHz. To be audible at this minimum level, a sound at 20Hz must be 80 dB (100 million times!) more powerful than a sound at 3 kHz.
Near the top, the curve at 100 phones is a fairly loud level. To sound equally loud at this level the sound at 20 Hz must be about 40 dB more powerful. This change in subjective level for different loudness levels means that music played softly will seem to be lacking in bass. For years pre-amps have come equipped with "loudness" controls to compensate for this. For me, part of "Hi-fidelity" means playing music at the same level it was originally played, so this is all academic - but interesting none the less.
An important characteristic of a sound system is the "sound image." An ideal system would create a vivid illusion of the location of each musical instrument. In designing a system it is important to understand, as well as current knowledge permits, how we locate the source of a sound. One thing that is clear is that the brain processes several different types of data to extract directional information. The data include:
- shape of the sound spectrum at the eardrum
- difference in sound intensity between the left and right ears
- difference in time-of-arrival between the left and right ears
- difference in time-of-arrival between reflections from the ear itself
A remarkable fact is that the pinna, the cartilage-filled structure surrounding the ear canal (commonly simply called the "ear"), is a vital part of direction sensing. Test subjects can be trained to locate sound using only one ear. But when the ridges of the pinna are gradually filled in, the ability is lost, in proportion to the filled in area. Apparently the brain uses reflections from the ridges of the pinna (19.4 kb) to determine direction. The head and pinna have a major effect on the sound that arrives at the ear. This effect is mathematically represented by a head-related transfer function (HRTF). There are files in the sound demo section where a monophonic sound source is processed with HRTFs to synthesize sound arriving from various directions. The full HRTFs contain both the difference in sound intensity, and difference in time-of-arrival. There are two other demo files where only one of these two differences are retained. When I listen to these files I perceive the apparent direction almost equally well with all three files, indicating that the brain has a remarkable capability of making good use of whatever information it gets.
The significance of the pinna reflection experiments for a sound system designer is that time delays on the order of 0.1 millisecond can effect sound imaging. Time delays between the left and right ear are on the order of 0.5 milliseconds, and are quite important. On the other hand, researchers have found that echoes in the range of 1 to 50 milliseconds are lumped together by the brain with the direct sound, so they are not actually heard as distinct echoes. Delays greater than 50 milliseconds are heard as echoes. My own echo research is described in the sound demo section, and you can listen to the results yourself. Echoes in the range of 25 to 100 milliseconds give a "cavernous" quality to the sound. What is commonly called an "echo," a distinct repetition of the original sound, only occurs for echoes of 400 milliseconds or longer. Echoes in the range of 0.1 to 2 milliseconds do cause changes in the apparent direction of the source.
A regular CD sampled at 44.1 kHz is theoretically capable of reproducing frequencies up to 22 kHz, which corresponds to a transient duration of .05 milliseconds. However, as discussed in a recent paper by Mike Story (e-mail mstory@dcsltd.co.uk to request a copy) the anti-aliasing filters required to record within this band cause the transients to be blurred, in effect smearing the ability of our ears to distinguish direction. Mike reports that in listening tests 96 kHz recordings provide notably better spatial resolution. In the Handbook for Sound Engineers Steve Dove says anti-aliasing filters "....exhibit serious frequency dependent delay and convoluted frequency/phase characteristics... leaving mangled audio in their wake". He also advocates sampling around 100 kHz, and says the result is a more open and spacious sound. Humans perceive left-right direction more accurately than up-down direction. Presumably this is due to the fact that we generally move in two dimensions along a more-or-less level surface. All of this information is important for the sound system designer, particularly regarding the control of sound diffraction and reflection, both of which can muddle the sound image.
Distortion is a commonly accepted criterion for evaluating high-fidelity sound equipment. It is usually understood to mean the tones in the reproduced sound that were not present in the original sound. An ideal sound system component has a perfectly linear response. This means that the ratio of the output and the input signal magnitude is always exactly the same, and the relative phase is constant, regardless of the strength of the signal. For a non-linear response (anything other than a linear response), distortion will occur. It is commonly categorized as total harmonic distortion (THD) and intermodulation distortion. Harmonic distortion means that a pure 1000 Hz input tone results in spurious outputs at 2000 Hz, 3000 Hz, and other integer multiples of the input frequency. Intermodulation distortion means two input tones at 1000 Hz and 100 Hz result in spurious outputs at 900 Hz, and 1100 Hz, among others.
The audibility of phase distortion is controversial. Some loudspeaker manufacturers, such as Dunlavy (apparently now out of business), cite flat phase response as a significant feature of their products. There is no question that under some artificial circumstances phase distortion is audible. Further discussion on the interesting topic of phase audibility can be found here.
So called "Doppler" distortion is produced by the motion of the loudspeaker cone itself. This creates some harmonic distortion, but the most significant effect is intermodulation distortion. This class of distortion can only be reduced by reducing the cone motion. A large surface, such as the membrane of an electrostatic speaker, will produce very little Doppler distortion. See the analysis for a piston in a tube for technical details.
Also see the discussion above on "clipping."
Everest quotes research indicating that amplitude distortion has to reach a level of 3% to be audible. However this varies greatly depending on the distortion harmonic products, and on the sound source. More on this below. Good CD players, amplifiers and pre-amplifiers typically have distortion levels of 0.1% or less. (Tube amps typically have higher distortion). Loudspeakers are the weak link regarding distortion. It is hard to even get information on loudspeaker distortion since it looks embarrassing compared to the values advertised for electronics. I measured 2nd and 3rd harmonic distortion of my sound system end-to-end using my CLIO sound measuring system. Since speaker distortion dominates, this is essentially a measurement of speaker distortion. The measurement was made using one speaker; with two speakers the distortion would be the same, but the SPL levels would increase 6 dB for the two lower frequency bands, and 3 dB for the upper bands. The entire measured distortion curve at the higher power level is shown in the section on final system measurements.
Measured harmonic distortion
Frequency
|
80 dB SPL
|
86 dB SPL
|
92 dB SPL
|
20 - 40 Hz
|
6%
|
8%
|
10%
|
100 - 200 Hz
|
1%
|
1%
|
1%
|
1000 - 5000 Hz
|
0.4%
|
1%
|
1.5%
|
5 - 10 kHz
|
0.14%
|
0.25%
|
0.44%
|
Distortion is universally considered to be bad, and it is perhaps not generally realized that musical instruments introduce overtones that have similarities to distortion. I imagine most music lovers are aware that all musical instruments produce a fundamental tone (the "note"), and a series of overtones. The overtones are at frequencies higher than the fundamental tone, and give the sound a rich quality not possessed by a pure tone. Overtones are generally harmonics (integer multiples) of the fundamental frequency. The relative strength of the various harmonics gives the instrument its characteristic sound. You can hear a comparison of a real piano note [42kb] and a tone {42kb] with the same fundamental frequency, but lacking in overtones. There is also additional description of the spectrum of this note.
The ear is not perfectly linear and produces distortion. A short discussion of the non-linear behavior of the ear can be found in a separate section. Finally, air itself is non-linear, and harmonic distortion grows steadily as a wave propagates (see plane waves in the physics section). This is usually a very small effect, but can be significant in the throat of a horn speaker.
The subject of sound quality is not at all clear-cut. Even though tube amplifiers have higher measured distortion, a lot of knowledgeable people swear that they sound better. I finally dove into this subject in August 2006. I can clearly hear THD at 0.5% for a pure 440 Hz tone and the type of harmonics produced by a typical solid-state amp; for the type of harmonics produced by a single-ended triode amp I could not detect distortion until it reached a level of 10%. This amazing difference is covered in detail in the section on amplifier distortion. For music samples the difference is not quite as big, but is still quite significant. Many people have come to the conclusion that THD is a terrible way to judge amplifier quality, and I totally agree. Norman Koren, an advocate of tube amplifiers, has posted a very interesting commentary on the subject of distortion and the effect of feedback.
Suscribirse a:
Entradas (Atom)